by Johnny Drain
This series is about oxidation, rancidity, and aging butter. In Part 1 I gave some background about butter, rancidity and the cultural context for eating aged butter. In this second part, I’ll explore the science of oxidation in fats and the safety of eating them. I’ll then describe the results of my work on culturing butters with unusual sources of bacteria in Part 3 and on aging butters in Part 4.
In Part 1 of this series, we examined how rancidity, culturally speaking, is rather poorly defined: foodstuffs can be seen as rancid depending on historical precedent and context, even though they may involve similar or identical chemical processes in foodstuffs we regard as delicious. From a scientific perspective, rancidity can be defined a little more strictly, though we will see that discussing the science of rancidity, oxidation, and their relationship to each other can still become unclear. In this post, I will outline some of what we know of the science behind rancidity in fats and examine whether such fats are safe, and indeed where they already enter our diet.
‘Rancidification’ can be broadly defined as the development via chemical transformations of new, sensory-active, often hedonically-negative compounds that don’t play a significant role in a food product’s expected/desired sensory profile. More specifically, with respect to fats and oils, it refers to the decomposition of triglycerides by hydrolysis and/or oxidation into free fatty acids, which can then break down further into a wide array of flavourful compounds [1]. The majority of this second post will be devoted to digging into the technical details of this definition, which we can use to start to understand why old butter is deemed ‘rancid’ but blue cheese is not, even though the same chemical processes may have taken place in both.
In rancid fats, the characteristic tastes and smells come primarily from the short- and medium-chained free fatty acids, whose carbon chains contain 12 or fewer carbon atoms (those with more are generally tasteless). Of these, the acrid-tasting, sweet- and goaty-smelling butyric acid (CH3CH2CH2-COOH, i.e. 4-carbon free fatty acid) is the primary culprit (Figure 1). In small quantities it can make positive contributions to flavour profile—it is found in sheep, goats and buffalo milk, Parmesan and mozzarella, and kombucha—but in larger concentrations is offensive: it can be used to make stink bombs and is the primary smell in human vomit.
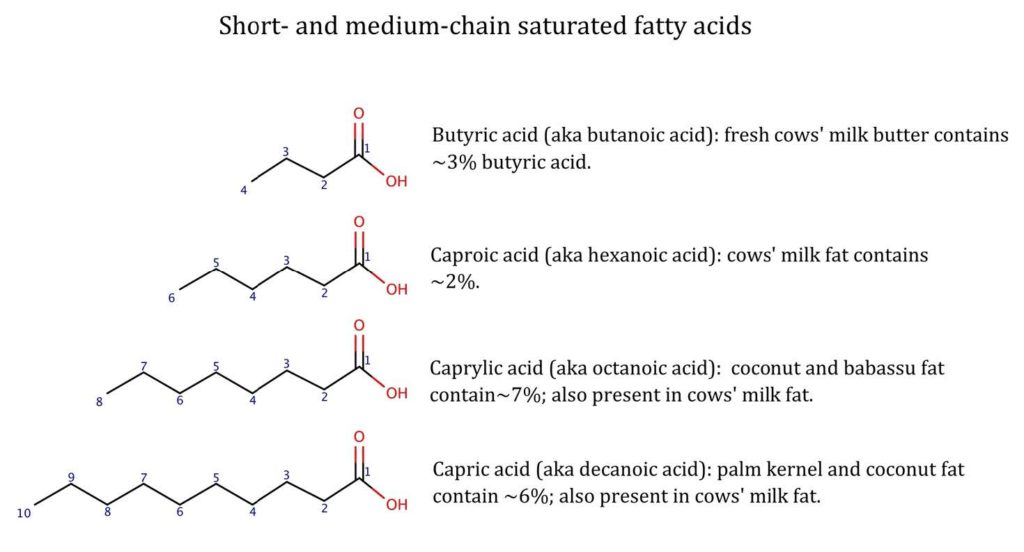
Around 4% of fresh butter is made of a triglyceride of butyric acid, and when this breaks down the butyric acid is liberated and we can smell its sickly, caprine smell. Other responsible free fatty acids include caproic acid (6 carbons), caprylic acid (10), and capric acid (12)(Figure 1). The shared goat-related Latin stem gives you an idea of what they smell like!
Other compounds that contribute to rancid flavours in degraded fats include acetic acid, peroxides, alcohols, aldehydes and ketones. There are three main pathways responsible for the breakdown of fats and for the formation of these compounds that contribute to rancid flavours. Their principal mechanisms are well-characterised, although their precise effect on flavour are still not fully understood.
Oxidation
The most important rancidity reaction pathway in butter is oxidation (Figure 2). Oxidation of fat involves cascades of free radical reactions that cause the C-C double bonds of unsaturated fatty acids to break. This releases peroxides as primary reaction products, and subsequently alcohols, aldehydes and ketones, as secondary reaction products, which can then degrade further into various volatile products. The primary oxidation products have little or no effect on taste and smell, but the subsequent reaction products do. Oxidation reactions can be initiated by oxygen in the air or light (photo-oxidation), and are catalysed by metals; they are chain reactions that can propagate in the dark once started—so butter can turn rancid even if stored in the fridge or a cloche, if it has been previously exposed to light or oxygen.
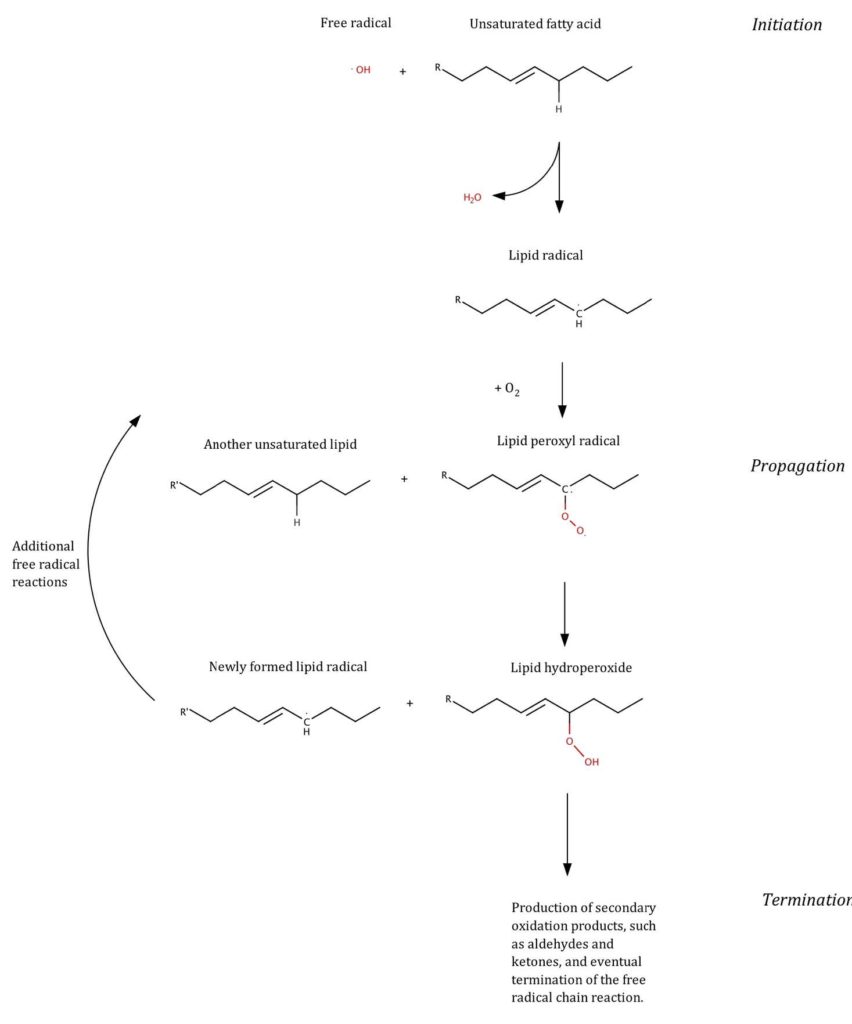
Hydrolysis
The second critical reaction pathway that causes the degradation of fats involves hydrolysis by water and heat of the -O-alkyl ester bonds of a triglyceride to liberate free fatty acids, such as butyric acid, from the glycerol backbone (Figure 3). Polymerisation of the free fatty acids can then lead to the gummy deposits found on utensils used to store or cook with used cooking oil.
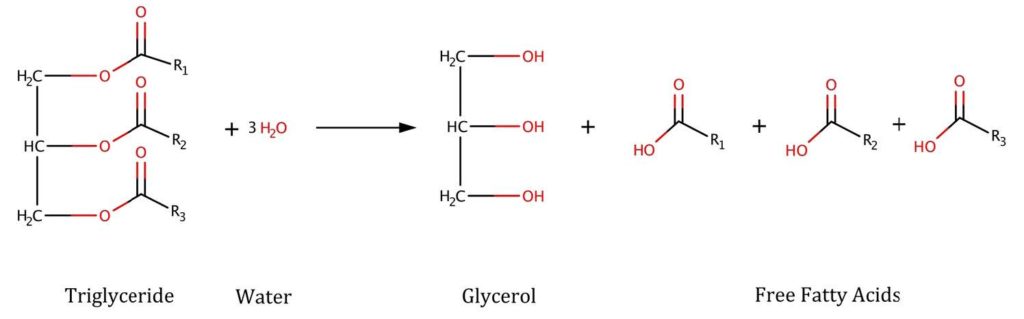
Enzymatic lipolysis
Spoilage of fats can also be caused by microbes, or certain enzymes—lipases—they produce. As mentioned in Part 1, many of the compounds found in rancid fats, and the processes that form them, are critical to flavour development in aged cheeses. For example, moulds that are introduced to blue cheeses, such as Penicillium roquefortii in Roquefort, produce lipases that break down triglycerides via hydrolytic reactions to create short-chain fatty acids, leading to spicy and peppery flavours. Enzymatic lipolysis also plays a key role in flavour development of hard cheeses such as Fiore Sardo and Pecorino, in which the lipase comes from the rennet paste used to initially coagulate them [2]. At this point, it’s probably also worthwhile dwelling on the primary chemical difference between cheese and butter: cheeses contain significant amounts of protein, whereas butters don’t. Therefore, proteolysis (the breakdown of milk proteins such as casein into amino acids and then volatile compounds) is a significant flavour formation pathway in cheese, but not in butter.
Terminology!
Now that we have established the chemical processes that can lead to rancidity, it is worth returning to the issue of terminology, specifically the distinction between the words ‘rancid’ and ‘oxidised’.
The first issue is that, in common parlance, they are sometimes used interchangeably. This conflation is not wholly incorrect: it is possible to have rancid fats that have been degraded entirely by oxidative processes, and to have oxidised fats that are rancid. However, this is not always the case: it is possible to have a fat that is rancid but that has not been oxidised (if it was degraded purely via hydrolysis), and to have a fat that is oxidised but not generally deemed ‘rancid’ (if only primary oxidation products, which one cannot taste or smell, have been produced, or, for example, as was highlighted in Part 1 in cases of chocolate- and toffee-making, if the oxidation leads to added desirable complexity of a flavour profile).
The second issue is whether positive or negative attributes can or should be ascribed to either of the two terms. In common and scientific parlance, both ‘rancid’ and ‘oxidised’ typically have mostly negative connotations, but long-standing examples can be found where they are used to express a desirable, positive character (most notably in the world of wine—’rancio’ with respect to e.g. sherries and cognacs, and ‘oxidised’ with respect to many natural wines).
To make sense of this blurry field, the key point to grasp is that the two words describe processes and properties that are non-binary. Many archetypally ‘rancid’ and ‘oxidised’ flavour compounds make important, hedonically positive contributions to flavour profiles in quantities below some (ill-defined, contextual) threshold, e.g. 3% butyric acid in fresh butter. It’s only if they are produced in larger quantities, that we might in common speech start terming the product in which they are found as ‘rancid’ or ‘oxidised’. (As an aside here, it is interesting to note that the descriptor ‘oxidised’ has entered, to some extent, the popular vernacular but the descriptor ‘hydrolysed’ has not and remains the preserve of the world of science.)
It’s not easy to distill the complexity with which these terms are used and understood into something simple, but we’ve devised a Venn diagram-like framework, shown in Figure 4, to try to clarify their interrelations. This diagram also helps to clarify the central concept behind the project: can we create a butter analogue of blue cheese, ‘blue butter’, which uses oxidation and/or hydrolysis to develop flavours that are a) intended/expected/desired and, therefore, b) not perceived as ‘rancid’ or ‘unpleasant’.
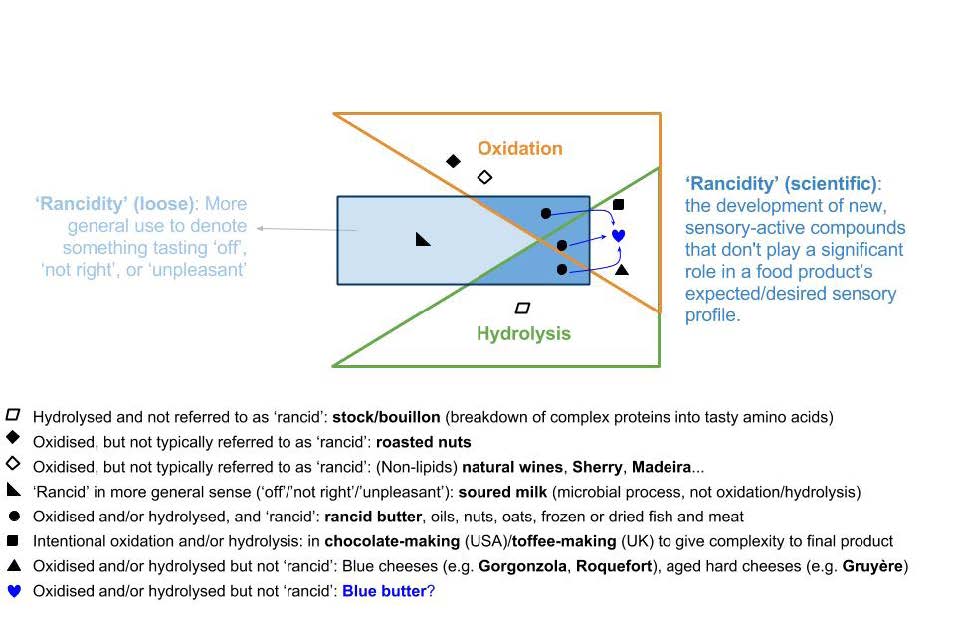
Factors affecting rancidity
The proneness of butter to turn rancid is dependent on what the butter is made of, how it has been made and how it is stored. The variables include: exposure to oxygen, light, and heat; the presence of antioxidants, microbes, and catalysts (e.g. metals such as iron and copper, which kitchen implements might be made of); the water and salt content of the butter; and the saturation of the fats in the butter.
For example, the oxygen in semi-solid room-temperature butter can move through the butter more freely (has higher mobility) than in colder, more solid butter: room temperature butter will therefore, all else being equal, oxidise more quickly than refrigerated butter. Furthermore, butters with higher concentrations of water can spoil more quickly, predominantly because free metal ions in the water can catalyse oxidation reactions [3].
Conversely, butters with a higher level of saturated fats will be more stable with respect to rancidification. Similarly, butters that contain more antioxidants—occurring naturally in the diet of the cows or added at some point during processing—remain stable for longer.
Antioxidants typically scavenge oxygen by being sacrificially oxidised, thus preventing the formation of primary oxidation products. Once antioxidants are exhausted, the level of primary oxidation products increases. However, these are not detectable to the human palate. Therefore, how quickly something turns rancid can be deceptive. For example, two samples, one with a much higher concentration of primary oxidation products, may taste, look and smell the same. It has also been found that some antioxidants—most notably for this project, some species of seaweeds found on the Danish coast—serve the extra function of actually reducing secondary oxidation products to their original oxidation states [4].
Modern manufacturing processes for many foods involve addition of a panoply of synthetic antioxidants, each with various strengths and weaknesses: some common ones include propyl gallate, BHA and BHT. However, traditionally, many naturally occurring antioxidants have been used to preserve fats. For example, studding a ham with cloves, which contain antioxidative phenolic compounds, serves to add flavour but also protect its fats from free radical oxidation; and slippery elm bark has been used to preserve bear fat [5]. Furthermore, oats—a good source of phenolics— might serve an antioxidative effect in the double cream cheese Caboc (reputedly Scotland’s oldest cheese, dating from the 15th Century), which is rolled in toasted oats.
With the advent of more rapid processing and distribution methods, modern butters and oils typically reach consumers in a less oxidised state and/or with more antioxidants intact. For example, in many places olives were traditionally harvested in batches and stored, perhaps even for months, until enough had been collected to press [6]. As a result, oxidation and fermentation would take place, and polyphenolic compounds, which impart the oil with spiciness and bitterness, would be sacrificially consumed. (Leaving them to rot slightly was also said to facilitate extraction of the oil, leading to greater yields.) The resulting oil would be milder and smoother than the ones that are made today, in which olives tend to go from tree to oil in under 12–24 hours.
Is eating ‘rancid’ or oxidised fats bad for you?
There have been a number of alarming claims about the harmfulness of rancid fats, linking them to, among other things, cancers.
However, much of the research we found cited alongside such claims has been extrapolated or is only tangentially relevant, e.g. experiments performed on animals in which, in order to produce measurable levels of adverse effects, much larger amounts (in relation to body weight and lifespan) than would be encountered by humans were used. Food: The Chemistry of Its Components highlights this fact, and adds that “the possibility that lipid oxidation products are toxic to humans remains unresolved” and “[despite claims to the contrary] there is little evidence at the present time to suggest that oxidized fats do cause cancer in humans.”[7]
Furthermore, McGee’s On Food and Cooking reassures us that “rancid fat won’t necessarily make us sick, but it’s unpleasant”, [8] and, as mentioned, the processes through which we make some cheeses, and the compounds these processes produce, are analogous to those that cause rancidity in fats.
Therefore, in essence, the answer to the question is: no, not in the amounts one might typically consume them as part of a balanced diet.
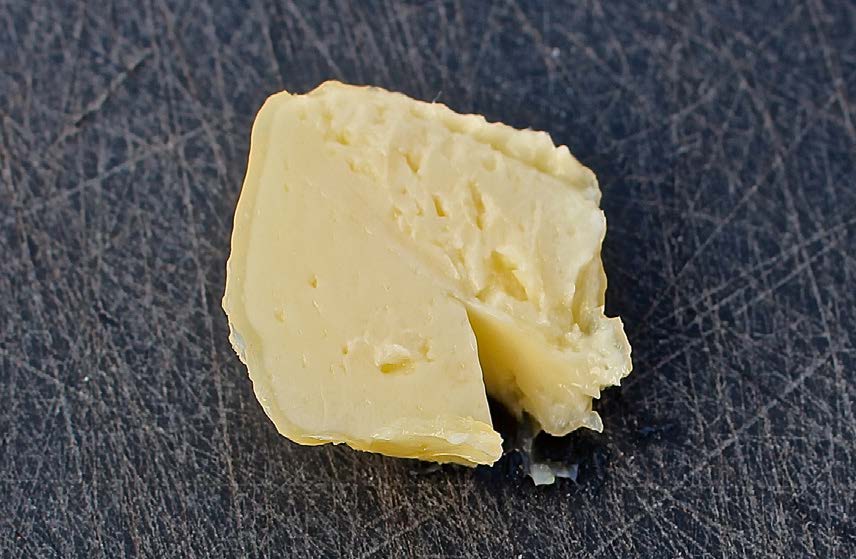
Summary
Given that the chemical processes responsible for rancidity in butter, and the flavours and aroma compounds they produce, are common to and characterise many much-loved cheeses, and that, furthermore, the perception of rancidity and oxidation in foods and fats are culturally elastic and context-dependent, the central hypothesis of this research was that we could make butter in which some mildly ‘rancid’ character added richness and complexity to its flavours, thus enhancing its gastronomic qualities, uses and value.
For example, could we prioritise one rancidity pathway over others to produce a desired flavour profile? Could we stabilise a mildly rancid butter in a given state using an appropriate combination of antioxidants or storage conditions? Taking inspiration from existing products—such as Morocco’s aged smen and blue cheeses that undergo significant lipolytic flavour development—could we reframe rancidity in butter as a positive quality?
In the next two instalments I’ll detail the results from our tests that explored whether this was indeed possible: this involved heaps of bacteria sourced from unusual places, an interminable obsession with pH readings, and a lot of cream.
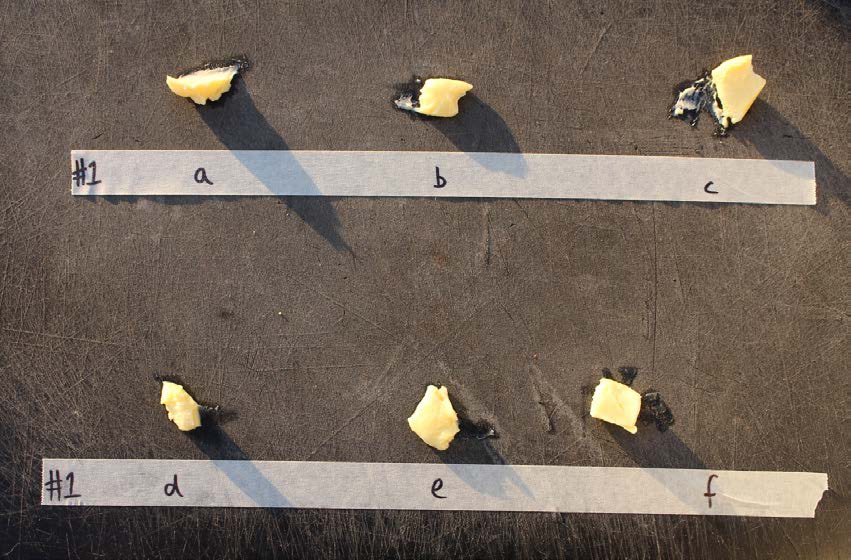
References
[1] Allen JC. & Hamilton RJ. (1994). Rancidity in foods. London: Blackie Academic.
[2] Fox, PF. (2004). Cheese: Chemistry, physics and microbiology: Volume 1: General aspects, Elsevier: Academic Press. 60
[3] Saxby MJ. (1996) Food taints and off-flavours. Boston, MA: Springer US. 176.
[4] Farvin KS. & Jacobsen C. (2013). Phenolic compounds and antioxidant activities of selected species of seaweeds from Danish coast. Food Chemistry. 138(2-3), 1670–81.
[5] Min DB. & Smouse TH. (1985). Flavor chemistry of fats and oils. Champaign, IL: American Oil Chemists’ Society. 155.
[6] Vossen, P. (2007). Olive oil: History, production, and characteristics of the world’s classic oils, Hort. Science, 42(5), 1093–1100.
[7] Coulate TP & Blumenthal H. (2009). Food: The chemistry of its components. Cambridge, UK: Royal Society of Chemistry. 122–123.
[8] McGee H. 2004. On Food and Cooking: the Science and Lore of the Kitchen. New York: Scribner. 204.